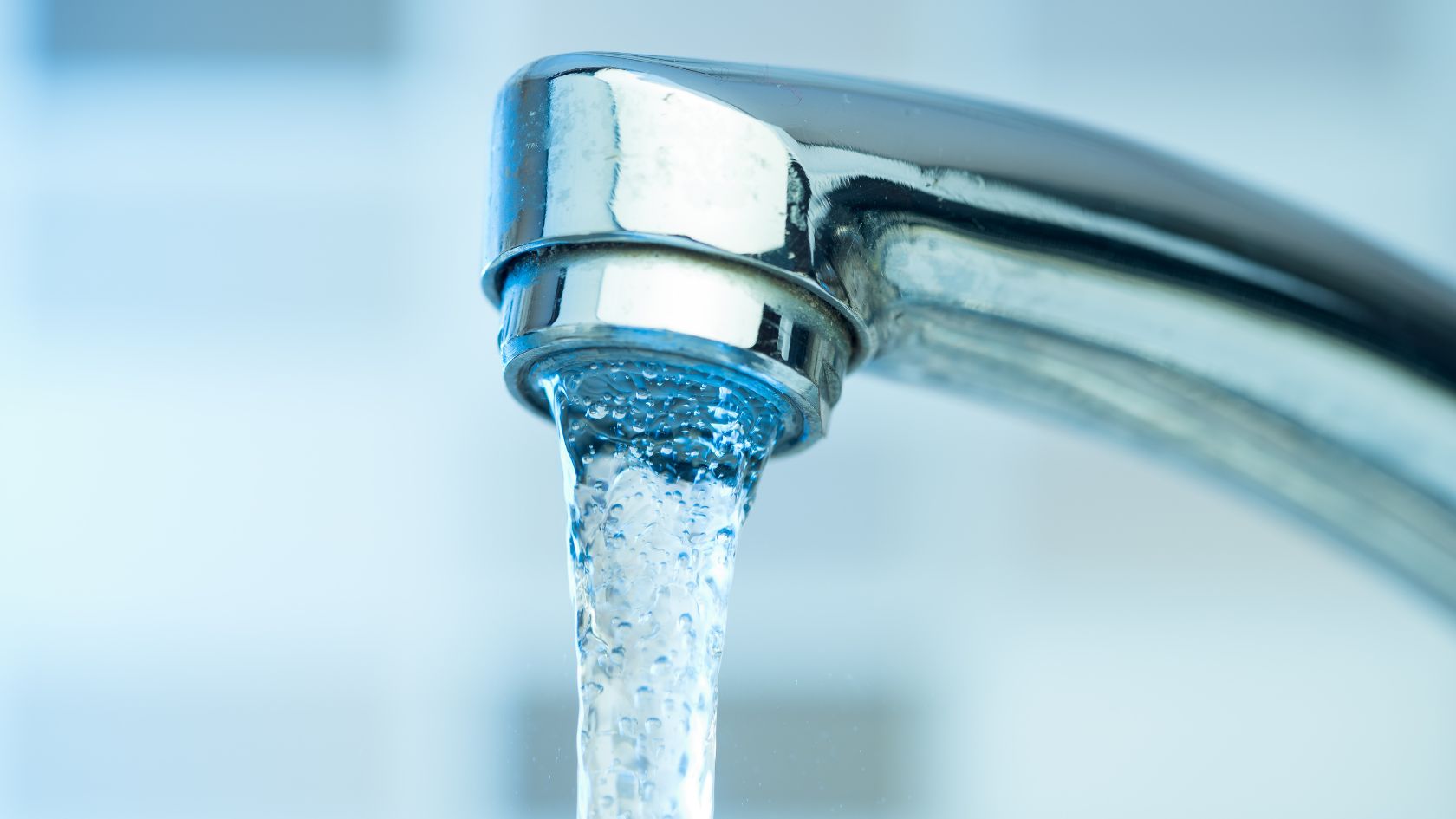
Urban Water Consortium
The Urban Water Consortium was established in 1985 to connect urban water managers with scientific research and information and technology transfer. Today, urban water managers from 12 of North Carolina’s most populated areas are members of the Consortium, and they continue to connect with one another, as well as researchers and experts, to advance drinking water and wastewater management across the state. WRRI serves as the main facilitator, arranging quarterly meetings and providing grant administration and management for research projects supported by the Consortium.
Operations
Member utilities contribute annual dues that are used to support research to enhance management and understanding of water resources and wastewater management. Utilities benefit from quarterly meetings and have the opportunity to share, learn and discuss common concerns related to current and future drinking water and wastewater management needs, or changes and and adapting to operating and regulatory requirements. The UWC considers research proposals that are submitted as part of WRRI’s recurring Request and For Proposals (RFPs), as well as through RFPs developed in collaboration with the UWC. We welcome researchers to share their ideas for utility-related research. Please contact Kaitlin Tucker to discuss your ideas.
Priorities
The UWC research priorities in drinking water, wastewater and water infrastructure fall into the following categories, though the group will consider research proposals on other issues of importance to water utilities in North Carolina.
Water treatment and management
What are innovative techniques and methods in biosolids treatment and management, especially as they relate to land application vs. incineration? How can carbon emissions related to alternative biosolids treatment be better managed?
Water affordability
What factors into water affordability (beyond income) and can existing datasets be used to conduct analyses on utility-specific affordability? Can models be developed to investigate water affordability in NC?
Emerging contaminants
What is the distribution and concentrations of emerging contaminants (including PFAS) in our water supplies and who is at risk?
Climate change impacts on water resources
How will more severe droughts and changes in rainfall patterns and amounts impact water supply and planning? How will flooding and increased high intensity storms impact reservoir sedimentation rates? How will increased temperatures impact toxic algal blooms in water supply source waters?
Innovative processes
What/how can innovative processes and technologies be applied to NC utilities for water and wastewater treatment, plant operation, energy production, distribution systems, waste discharge management, potable and reclaimed water supply, and the repair, management and planning of infrastructure?
Comprehensive list of projects
To view a list of projects funded by the UWC, please visit the WRRI Technical Reports Repository where all final project reports are housed. Type “Urban+Water+Consortium” in the “Search for” box.
Members
Contact
To contact the UWC, email Kaitlin Tucker, WRRI coordinator for research and engagement: ktucker@ncsu.edu